Implementation
- Students need to practice reading and interpreting models encountered in instruction. Skills required for interpreting, using, and building models are not intuitive, but can be developed with practice and instruction.
- Time on task is a critical component for all types of model-based learning.
- It is important to incorporate deliberate modeling practice to allow students to acquire and use skills, and build on these skills through increasingly complex tasks. This can be done with minimal class time.
- Instructors should promote opportunities for students to reflect upon the nature of models. Specifically students could articulate the purpose of models and modeling in general or within a specific type of model.
- Instructors cannot assume that models transparently convey meaning for students. Students need guidance on how to read and interpret each new model they encounter, even if the only difference is in formatting. To promote effective model use and interpretation, instructors can:
- include multiple models for a single phenomenon;
- explicitly describe the strengths and limitations of each model being used;
- provide a lexicon or key of symbols with meanings (e.g., an arrow can mean change over time/distance, flow of information, energy/matter transformation, movement, interaction/dissociation, external action applied, change in scale, progression through a system, or input/output);
- choose, if possible, diagrams or animations that consistently attribute the same meaning to common style elements (arrows, symbols, colors, etc.).
- Modeling activities (especially model construction) are particularly well suited for small collaborative group work in the classroom.
- Modeling activities tend to be complex and/or ill-defined with multiple possible correct answers; these are features of tasks that are usually best accomplished by students in groups.
- Collaborative model construction allows students to cooperatively communicate what and why certain components are needed in a model and how those components work together for the function of a system.
- The Group Work Evidence-Based Teaching Guide can help instructors make choices supported by the literature in structuring group activities.
- Activities that include model interpretation or development can impart a high cognitive load on students and cause excessive demands on working memory, thereby negatively impacting learning. Tasks associated with high cognitive load include working across biological scales or levels of physical organization (e.g., moving between molecular and organismal scales to explain why a mutation results in a specific phenotype), or using/building diagrams/models that include multiple levels of abstraction. Instructors can address high cognitive load by:
- Scaffolding learning activities to build student understanding and skills over time and complexity.
- Providing sufficient practice and time for students to complete complex or multi-part modeling tasks.
- Demonstrating and allowing learners to build fluency and learn to organize individual elements into larger chunks.
Hobbs, F. C., Johnson, D. J., & Kearns, K. D. (2013) A deliberate practice approach to teaching phylogenetic analysis. CBE Life Sci Educ 12, 676-686. Modeling is a skill that requires development. The authors investigate deliberate practice concurrent with building understanding of the content specific vocabulary and concepts of modeling. Deliberate practice of tree-building included five assignments of increasing complexity. Very noteworthy is that the incorporation of deliberate practice in this study took very little class time, as the assignments were given as homework and in-class time focused on feedback in small groups and whole class discussions. The assignments were low-risk in terms of contribution to course grade and students were allowed to modify their answers based on class discussion, creating a supportive approach for skill development. Students were compared to previous courses using the same final exam multiple choice and short answer questions. The intervention group that received the deliberate practice did significantly better than the comparison group on phylogenetic questions but performed the same as the comparison group on the non-phylogenetic questions. Likewise, the intervention group improved significantly from a pre- to a post-concept assessment. This paper’s supplement and methods include copies of the assignment prompts and a detailed description of how the increasing complexity of the practice was constructed. These could serve as an examples to construct practice assignments for building tree or other models.
Bierema, A. M. K., Schwartz, C. V., & Stoltzfus, J. R. (2017) Engaging undergraduate biology students in scientific modeling: Analysis of group interactions, sense-making, and justification. CBE Life Sci Educ 16: ar68. This article explores how students use class time to build models in both an upper-level major and a non-major biology course. They use a scaffolded approach of model construction, allowing students to develop models that are consistent with the current theoretic understanding of a system. Recorded group conversations during these activities were evaluated for on-task and off-task activities, statements that are “sense-making”, and for justification of choices in model construction. The analysis found on average that students in groups only spent ~10% of the time discussing something not directly related to the model for activities that were between 30 to 80 minutes of class time. This sustained attention to the modeling activity was primarily on key mechanistic aspects of the mode instead of trivial details. Sense making statements communicated why certain components were needed in the model and how those components worked together for the function of the system. The amount of sense making statements were influenced by the number of scenarios (different versions of phenomena or processes where some aspect had changed) present in the activity. Therefore, having differences in the models helped students compare and contrast the situation and understand better how the system works. As there was little difference in student interaction between the two groups, these data support the use of groups for modeling activities in either majors or non-major courses.
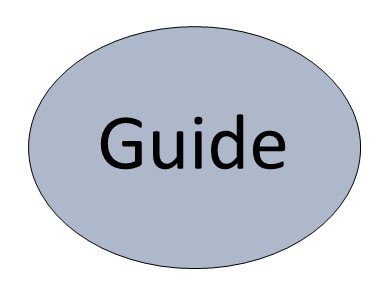
Wright, L. K., Cardenas, J. J., Laing, P., & Newman, D. L. (2018) Arrows in biology: Lack of clarity and consistency points to confusion for learners. CBE Life Sci Educ 17: ar6. Wright et al. performed a mixed methods study to understand the use of arrows and student interpretation of figures containing arrows. They created a codebook of arrow style and arrow meaning using one introductory biology textbook and used the codebook to examine a selection of figures from another textbook. They found that 52% of the over 1200 figures contained arrows with 230 different arrow styles, yet less than 1% of examined figures contained a key. Arrow style did not correlate with the conceptual meaning of the arrows (e.g., change over time/distance, flow of information, energy/matter transformation, movement, interaction/dissociation, external action applied, change in scale, progression through a system, or input/output). A large-scale survey investigated if arrow styles had intrinsic meaning to undergraduate students across a range of student experience levels. Most arrow styles had no inherent meaning to students with the exception of 3 arrow types. A majority of respondents classified an arrow with an X through the line to mean ‘inhibition of chemical action’, a gradient-shaded block arrow to mean ‘concentration gradient’, and multiple small block arrows to mean ‘multiple steps.’ The same survey showed that perceived arrow meaning could change depending on other arrows in the same figure, therefore interpretation is context-dependent. A semi-structured interview with undergraduate students found that all 14 participants were confused by the figures containing arrows (incorrect response, explicit confusion, or implicit confusion). The authors explain that experts have no difficulty interpreting figures even with confusing symbols because they are already familiar with the context. Students, being unfamiliar with the context, may find symbols like arrows more confusing than helpful, and may cause learners to experience cognitive overload trying to understand figures that contain a variety of arrow meaning and styles.
Offerdahl, E. G., Arneson, J. B., & Byrne, N. (2017) Lighten the load: Scaffolding visual literacy in biochemistry and molecular biology. CBE Life Sci Educ 16: es1. When students interpret or create visual representations such as models, it imposes a high cognitive load as the students are also developing fluency in disciplinary knowledge. Disciplinary knowledge is embedded not only in specialized oral and written language, but also in the representations which also contain conventions of display that codify knowledge. This paper develops a taxonomy to analyze the level of abstraction of visual representations that can be used to understand both textbook images as well as those published in the literature. The taxonomy divides diagrams into symbolic (abbreviations, formulas, names), schematic (abstract symbols, drawings, arrows), graph, cartoon (drawing or computer-generated image), and realistic. Offerdahl et al. discuss that in order for students to develop fluency in a specific topic, it requires practice with multiple representations, allowing students to make connections between them and to connect to disciplinary knowledge. Disciplinary fluency in canonical visual representations requires a multi-step process to decode and extract information, putting pressure on mental processing capacity. Levels of abstraction used in diagrams can present problems for students because the same concept can be represented by more than one image with varied degrees of abstraction and representations with multiple levels of abstraction are used for many concepts. Many textbook images contain multiple panels that often differ in their level of abstraction. Having students practice with multiple diagrams or models to explain a single concept is useful because no single representation can adequately convey a topic like enzyme inhibition. This paper recommends using a combination of representations with varying levels of abstraction so that students can gain a rich and holistic understanding of disciplinary knowledge.
- The goal of most model-based instruction is to develop students’ conceptual understanding, with limited attention paid to the process and best practices of modeling itself. As a result, there is limited research on how best to evaluate and provide feedback to students on their models or on the process of modeling itself.
- However, in reviewing the research that explores how modeling impacts students’ conceptual understanding in biology, we identify several themes about evaluation and feedback.
- Activities that engage students in the process of evaluating their own models (e.g., peer evaluation) may support the development of critical modelling skills while also helping students integrate biological concepts into a coherent framework
- Modeling is a skill and, like any skill, requires practice coupled with feedback. Instruction should provide multiple opportunities for students to model, ideally throughout the curriculum, providing feedback following each modeling activity.
- Student-generated models can reveal student difficulties, particularly as they relate to mechanistic explanations. Instructors can use student’s models to provide rapid, individualized, and specific feedback to promote both better modeling and conceptual understanding.
- Research from concept mapping provides several metrics that can be used to evaluate student-generated models, particularly concept models. These include quantitative metrics (e.g., number of links included, number of structures and behaviors) and more qualitative measures (e.g., model architecture).
Bray Speth, E., Shaw, N., Momsen, J, Reinagel, A, Le P, Taqieddin, R, Long, T. (2014). Introductory biology students’ conceptual models and explanations of the origin of variation. CBE-Life Sciences Education, 13, 529-539. Decades of education research document the challenges students from middle school through college have with learning natural selection. Model-based pedagogies, where students construct and evaluate conceptual models, may help students move past many of the learning difficulties associated with evolution by natural selection. In this study, Bray Speth and colleagues investigated student understanding of variation and origin of variation (i.e., mutation), key mechanisms underpinning natural selection. Data for this study were gathered from a large-enrollment (n=182) introductory biology. Feedback on content understanding and modeling was provided throughout the course, including following exams. In particular, students engaged in several model evaluation activities, evaluating sample models that were representative of student models. Evaluation activities were followed by full-class discussion, where the instructor summarized key ideas that emerged from the discussion. Students created and evaluated models as part of instruction and demonstrated their content understanding on assessments that asked students to generate case-specific concept models. Through analysis of student-generated models on the midterm and final exam, researchers found that students’ explanations of variation and the origin of variation improved over time and in response to instruction and feedback.
Dauer, J. T., Momsen, J. L., Bray Speth, E., Makohon-Moore, S. C., and Long, T. M. (2013). Analyzing change in students’ gene-to-evolution models in college-level introductory biology. Journal of Research in Science Teaching 50(6), 639-59. Models can help students organize their understanding of biological phenomenon and support students in building connections between biological concepts. When building models, students need practice and feedback on both biological content and the modeling process. Dauer et al. (2013), working in a large-enrollment (n=368) introductory biology course, explored student understanding of genetics and natural selection through student-generated concept (box-and-arrow) models. In particular, this research described how students’ models changed over time (quiz, mid-term, and final exam) in response to instruction and feedback. Throughout the course, students received feedback on their content understanding as evidenced through their models. Feedback guided students to identify and incorporate relevant biological processes into their models (e.g., labeling the arrow that links two structures). In class, students participated in peer evaluations of each other’s models. Through clicker questions and on-line rubrics, students received feedback on some of the most common errors found in students’ models (e.g., arrows lacked directionality, arrows weren’t labeled with a biological process, etc). Student models increased in complexity and correctness from the quiz to the midterm and remained stable to the final exam. In addition, students in the middle and lower GPA tritiles narrowed the gap with the higher tritile over time. These results have several implications for effective evaluation and feedback on student models. First, learning to model biological phenomena takes time and practice with frequent opportunities for feedback. Second, students need opportunities to revise their models in response to directed feedback on both biological content understanding and modeling skills. Finally, while students’ models do change in response to instruction and feedback, the process takes time and not all students progress at the same speed.
Johnson, SK, and Stewart, J. (2002). Revising and assessing explanatory models in a high school genetics class: A comparison of unsuccessful and successful performance. Science Education, 86, 463-480. Exploring the problem-solving approaches students use when approaching modeling problems can inform instructional practices. Johnson and Stewart (2002) use a qualitative approach to study how students approach model revision in a high school genetics module. Through analysis of conversations from two student groups (one successful and one unsuccessful), the researchers identified several strategies students used when attempting to revise a known model to fit new data. These included the ability to identify and process anomalous data, to evaluate tentative model revisions systematically, and to use mechanistic reasoning to check tentative models. These results reveal that students may struggle to think about the biological mechanisms underpinning a particular model. Instruction can use student-generated models and short explanations to uncover these challenges and provide just-in-time feedback for these learners. In addition, this research demonstrates the power of analyzing student conversations as they work with models, whether in a classroom or controlled laboratory environment.
Kinchin, I. M., and Hay, D. B. (2000). How a qualitative approach to concept map analysis can be used to aid learning by illustrating patterns of conceptual development. Educational Research, 42(1): 43-57. Instructors who use student-constructed models in their instruction face a challenge in effectively and efficiently evaluating those models. Research from concept mapping provides some insight into evaluation strategies. Historically, concept map evaluation focused on the number of valid links a student drew, the degree of cross-linkages and branching within the map, and the hierarchical structure of the map. However, these quantitative approaches were often tedious and time consuming, hardly practical for an instructor to implement. Kinchin and colleagues (2000) addressed this challenge through a qualitative analysis of concept map structure. Through an extensive and systematic analysis of student-generated concept maps (drawn primarily from middle school science classes), the authors identify three basic concept map structures: spoke, chain, and net. (Subsequent research by Yin and colleagues (2005) identified two additional structures, circle and line, to more fully capture the typology present in students’ concept maps.) Kinchin et al. then posit a progression in concept map structure as students integrate more concepts into their maps, from spoke to chain and finally net. This typology is straightforward, quick and easy to use, providing information on how a student has structured and restructured their understanding of a given concept. Although developed for concept mapping, this approach could easily be adapted to modeling, particularly concept models.
Cite this guide: Wilson KJ, Long TM, Momsen JL, Bray Speth E. (2019) Evidence Based Teaching Guide: Modeling in Classroom. CBE Life Science Education. Retrieved from https://lse.ascb.org/evidence-based-teaching-guides/modeling-in-the-classroom/